A journey with Damien Gasse, geologist
Interview with Damien Gasse, geological engineer at Seqens for the Mineral Specialties activity.
OUR EMPLOYEES ARE TALENTED
Hello Damien, can you start by introducing yourself?
I’m Damien Gasse, engineer in geology, now in charge of the Raw Materials department at the Novacarb plant in La Madeleine, near Nancy (France), for the Mineral Specialties activity.
The department’s role is to ensure the exploitation and delivery to the plant of the raw materials that are essential for our activity. Some raw materials, such as coke and anthracite, which are used as fuel in lime kilns, or ammonia, which arrives by tank car, are purchased and our role is solely to ensure their reception and processing at the plant. On the other hand, we are integrated on the raw materials that go directly into the production process of our sodium carbonate and bicarbonate: salt and limestone. We extract these mineral resources on two mining sites… which explains the presence of a team of geologists at Seqens!
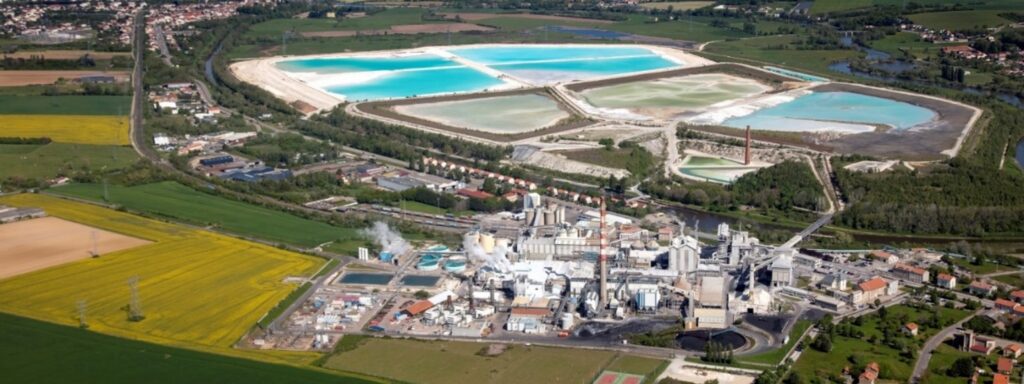
How did you get to Mineral Specialties?
I studied at the Faculty of Sciences and then at the School of Geology in Nancy. I specialized in Economic Geology, and particularly in exploration. My very first assignments were during an internship on gold exploration in Morocco and uranium exploration in Mongolia. These periods were decisive and confirmed my project. After graduating in 2007, I travelled quite a bit before returning to the region. I travelled across Africa for 4 years as an exploration consultant: Mauritania, Angola, Sudan, Saudi Arabia…
Exploration takes us further and further away: we often find ourselves in very isolated places, in the desert or in the forest, several days’ drive from the nearest town. If we add the exchanges and work with local people, the life of a geologist is full of surprises.
Backpacker! Is it a passion?
You could say so! I’m part of the “Jurassic Park generation” who dreamed of paleontology, so I have a natural penchant for exploration. Finally, my studies in mining geology have allowed me to satisfy this attraction for excavation and prospecting; instead of fossils, I simply look for other types of resources.
After those first years of field training, we set up our own service company, Afrex Geoservices, with a colleague, which had up to 6 geologists. Our great success was to identify and characterize a very large sedimentary phosphate deposit in the Republic of Congo.
Is this an event?
Probably; some people search all their life!
Can you explain us how to go about finding a deposit?
A client, who had been granted a license to explore towards Pointe Noire, in the coastal basin of Congo, suspected the presence of phosphate, a mineral that is essential for producing fertilizers. We analyzed the available bibliography and historical fieldwork. Decades of oil exploration and drilling have enriched the database of the national geological survey, in particular that of BRGM (Bureau de Recherches Géologiques et Minières) in France.
On this basis, and after discovering the first field showings, we launched an exploration program consisting of geochemical and geophysical analyses and then scout drilling when the first targets were identified. Once the contours of the deposit and its characteristics were understood, we spent several years drilling by tightening the drilling grid: first every kilometer, then every 500 meters, then every 250 meters, etc. Once the deposit is delineated, statistical methods are applied to quantify the resources. The finer the drilling grid, the better the classification of the resources, allowing them to be converted later into mineral reserves when a project feasibility study is produced.
That’s where my work stopped, on this site. After 5 years with Afrex Geoservices, it was also the right time for me to return to France. Joining Mineral Specialties, in my home region, was a chance after so many years abroad: I joined in 2016 as manager of the salt mining and effluent settling ponds at the plant. In 2017, my perimeter expanded with the addition of our limestone quarry and the reception of raw materials at the plant.
So it’s not a question of extracting from the finished product?
No! There are deposits of natural soda ash, called Trona, but they are rare in Europe. The largest deposits are located in Wyoming (USA) or, closer, in Turkey in the Beypazari region. Trona contains more impurities (metals…) than the synthetic sodium carbonate that we produce by mineral chemistry at the La Madeleine plant.
The sodium carbonate and bicarbonate (Na2CO3 and NaHCO3) production units are always built near a salt deposit (sodium chloride, NaCl). Together with limestone (calcium carbonate, CaCO3), these are the two basic mineral resources of the production process.
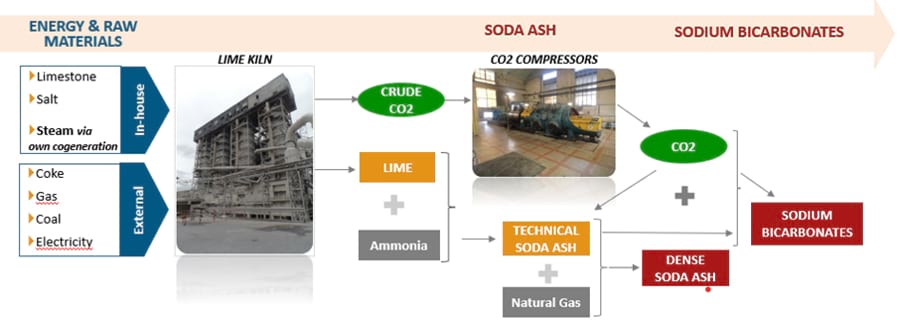
Let’s start with the limestone: where does it come from?
The one we exploit at the Revoi quarry, located in Pagny-sur-Meuse, comes from geological levels dating back about 160 million years, a period of the Upper Jurassic called the Oxfordian. At that time, in our latitudes, the region enjoyed a hot and dry climate. The sea is present in Pagny and there is a carbonate reef platform environment, with open wave environments and lagoon areas.
In this environment, marine organisms breathe, causing the precipitation of dissolved calcium ions (Ca2+ + 2 (HCO3-) ↔ CaCO3 + CO2 + H2O): carbonate sludge is deposited as limestone.
Frequently, other so-called terrigenous elements (silica, aluminium, iron) resulting from the erosion of the continents are found in these deposits, but the carbonate production is such in the area at that time that it fills the available space leaving no room for the accumulation of detrital minerals. These favorable conditions for sedimentary deposits allow us today to extract a very pure limestone, greater than 99% CaCO3.
Moreover, we find a large quantity of rock facies and fossils, which is a delight for sedimentologists and paleontologists at the University of Lorraine!
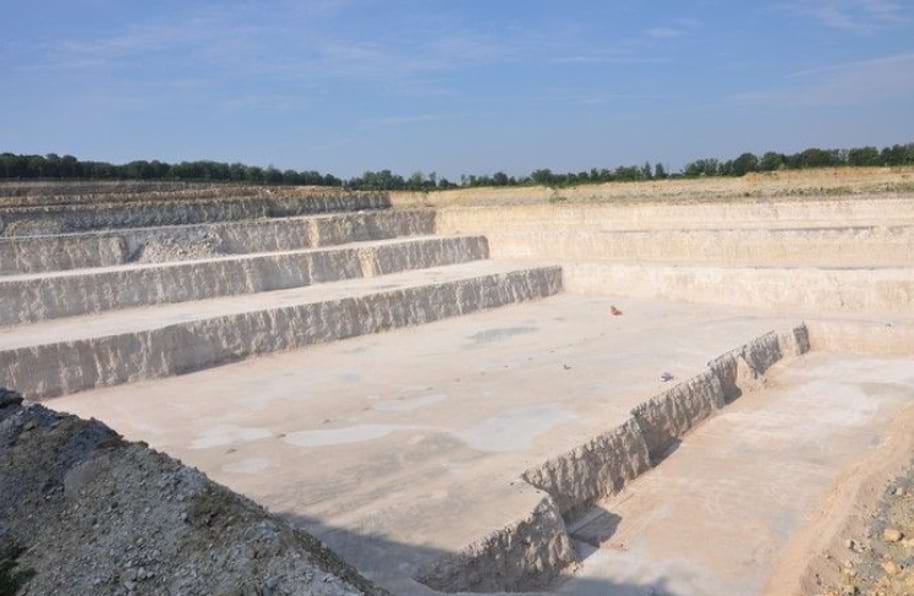
Operating techniques are being modernized?
Yes: 1.5 million tons of limestone are extracted each year, a large part of which is fed into the lime kilns that produce the CO2 needed for synthesis. This requires a large amount of mechanical work and mobilizes a lot of quarry machinery. Optimizing practices therefore makes sense.
Surveying the site by drone has become standard practice: the images obtained are georeferenced and processed by photogrammetry to obtain digital surface models. From there, we can access the volumes of material shot down. UAVs (drones) can also be used to map the quarry faces and their roughness, which allows us to adjust explosive charges and go further in the optimization process. Such methods are still under development, but we use them occasionally.
We have also initiated satellite tracking tests of the devices: this provides us with statistical data on mucking times and on device cycles in general. The analysis of this data allows us to identify the stopping points and steps that cause slowdowns, whether in the quarry or on the processing facilities, such as crusher throughput, for example. These are the beginnings of a “learning system” approach to identify the blocking points.
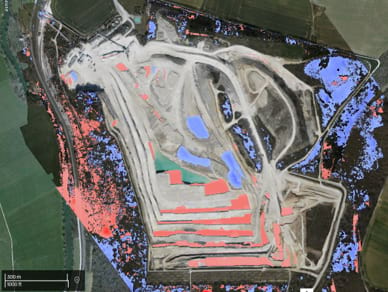
What about the exploitation of salt, the second raw material?
Our Lenoncourt mining tenement must supply an average of 350 m3/h of saturated salt brine, continuously, 24 hours a day. This represents approximately 850 Ktons of rock salt equivalent per year.
The only buffer tank at the La Madeleine plant offers only about 15 hours of autonomy in the event of a supply stoppage, so the stakes are high.
What is the history of this salt?
We have to go back a little further in time, to the Upper Triassic, some 220 million years ago. The Atlantic Ocean had not yet opened and there was a sea, called the Germanic Sea, in the East.
At that time, part of eastern France was on the edge of this sea, Lenoncourt was located in a depression with a sill overlooking the sea. When the sea level rises, the depression fills up with salt water; when the level falls, the basin is no longer fed: the water stagnates, evaporates and the NaCl is deposited. This layer was then covered by other layers, marly and clayey.
The Lenoncourt salt deposit is not very thick, only about 150 meters (salt layers in Northern Europe are found to be up to 1 km thick) and we only mine the top three layers, which totalize about 80 meters, but it has the advantage of being close to the current surface, at a depth of about 220-300 meters. The salt deposit is even closer to the surface in the surrounding valleys and that is why its exploitation began in the middle of the 19th century.
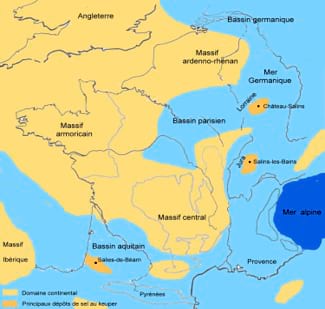
How do you extract it?
Salt is extracted by in-situ dissolution, the “solution mining” method, which uses mining boreholes called wells linked together by dissolution corridors and caverns within the salt massif. Some wells introduce fresh water and others extract brine.
Historically, the wells were connected to each other by hydro-fracturing, i.e. injecting water under very high pressure. The pressurization of an incompressible fluid caused the salt layers to fracture and open up along strata boundaries, allowing the connection between the wells to be forced. Once the connection was established, fresh water circulations created salt caverns and transformed the fresh water into brine. This technique had a big disadvantage: although it was possible to measure the quantity of salt extracted, it was not possible to know the shape of the sculpted cavern or the vertical evolution of the dissolution within the massif. As a result, the extraction was intensive, the salt depletion rate was often too high and the overburden lost its stability, leading to ground collapses: we still have to trigger collapses at our mining site as a result of cavities created several decades ago.
Here, too, we use drones to monitor the topography of the ground.
The arrival of new technologies such as echometry using sonar probes to determine the shape of the caverns made it possible to improve the method from the 1990s onwards.
The technique of connecting the wells to each other was also greatly improved. From now on, the method is based on controlled dissolution, forming a preferential passage. In addition to fresh water, compressed air is injected to form a protective blanket at the cavern roof. The fresh water, which has a natural tendency to dissolve the roof of the cavity due to its lower density, is then forced to spread to the sides, forming a plate-shaped cavity. By drilling a well every 50 meters and repeating the operation, the plates are connected to each other to create a controlled shape: this is the coalescence phase, which completely replaces hydro-fracturing.
In the end, only the first and last wells are set up for injecting fresh water and extracting brine. During this exploitation phase, other probes allow us to evaluate the thickness of residual salt. We are working with the Ecole des Mines de Paris to establish geo-mechanical stability models and to determine the thickness that must be preserved to ensure the overburden stability and to avoid any ground collapse phenomena.
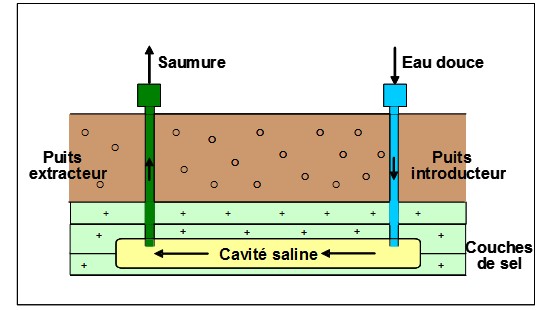
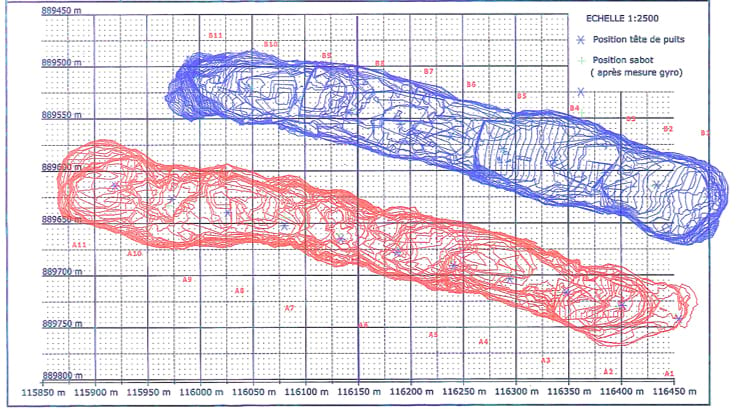
These are substantial projects.
Yes, the development of our next salt cavern requires 16 wells, it requires important investments, in the range of 300 k€ per well, but also a lot of time. This new cavern is part of the development of a new exploitation area which will eventually include about a hundred wells and 5 large caverns.
Obtaining the permits to start new mining works takes several years. It then takes 6 months of drilling for each new track, followed by about 2 years to coalesce and create the proto-cavity before it can be mined. Several people from my department work on these projects and we call upon external service providers as well as Novacarb’s design office for some aspects specific to these new projects.
The lifespan of a salt track depends on its length, in general the operation lasts 2.5 to 5 years, so we have to renew the operation regularly if we want to perpetuate the access to the resource.
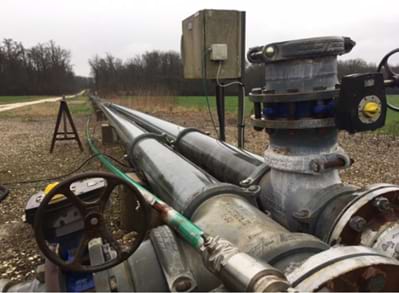
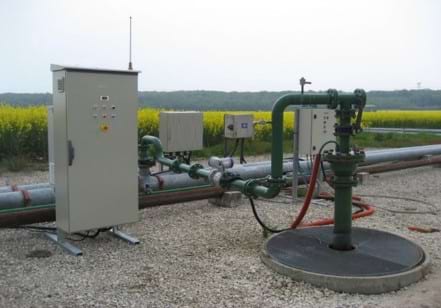
Why are the authorization phases so long?
Because the environmental aspects are paramount for our projects, and this requires lengthy studies.
We have installed, and continue to develop as our operations expand, a groundwater monitoring network based on piezometers to monitor aquifer levels and check that there is no connection with our salt caverns that would result in excessively high chloride levels.
But environmental issues are also played out on the surface! Part of the exploitation is located in the forest, some of it must be cleared to install the wells. For each new project, we therefore carry out inventories for an average of 2 years to measure the initial condition of the fauna, flora and habitats so that we can then assess the impact of our project on biodiversity and propose the implementation of avoidance, reduction or compensation measures if necessary.
We also work closely with ecologists’ associations in order to propose accompanying or monitoring measures. This is the case, for example, for chiropterans (bats), for which we have set up special lodgings in order to develop their presence on our site.
It happens that some species settle down. A Red Kite has installed its nest on our exploitation. It is a large migratory bird of prey, endemic to Western Europe. In France, the species is classified as endangered, so the tree on which it has taken up residence is well preserved and under surveillance!
Let’s save the Red Kite, then!
In conclusion, how do you feel about being a geologist in a Group populated by chemists?
Quite well! The job requires a lot of technical skills with a multidisciplinary approach to deal with all aspects of new projects. We intervene upstream of the manufacturing process with the supply of raw materials but also at the end of the chain with the management of effluents from the process in settling ponds. The tasks are diverse and the stakes are different from one site to another, but the common objective of all the sectors making up the Raw Materials department is of course to ensure that the plant is able to produce.
Major investments are required to continue mining our deposits. The Mineral Specialties BU has taken this factor on board and we are increasingly involved in discussions with other departments.
Thank you, Damien, a journey from beginning to end!