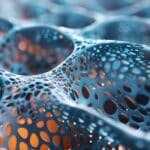
Polymeric scaffolds used in regenerative medicines
Regenerative medicine is an emerging interdisciplinary approach to repairing or replacing damaged or diseased tissues and organs. Humans can naturally regenerate some tissues, including the liver, which if damaged due to disease or injury, can grow back to its original size and function, though not its original shape. However, many other human tissues do not regenerate, and the purpose in regenerative medicine is to find ways to promote tissue regeneration in the body, or to engineer replacement tissues.
Tissue engineering and overall regenerative medicine, involve complex processes that often require combining multiple strategies. These include developing multifunctional materials [1] (scaffolds), delivering growth factors or biochemical signals, applying cell and gene therapies, modulating the immune system, and using external stimuli like electrical or magnetic pulses. Together, these approaches have the potential to meet the growing demand for organ replacements.
Materials used in these approaches, range from metals and ceramics to natural and synthetic polymers as well as their microcomposites and nanocomposites.
Biocompatible scaffolds
To promote tissue regeneration and reconstruction, scaffolds or structures made from polymeric materials typically undergo hydrolytic degradation, yielding non toxic products that the body can metabolize. The degradation time and rate vary depending on the polymer type, ranging from a few weeks to months or even years. Biodegradable polymers, both natural and synthetic, are more biocompatible than solid materials
The application of polymer scaffolds in soft tissue canal regeneration involves several key elements, including the proper design and fabrication of the structure, collaboration with stem cells, and processes that enhance cell growth. During regeneration and reconstruction, damaged tissues need structural support to promote stem cell proliferation and migration.
Polymeric materials, both natural and synthetic, are used in implants and prostheses. Their structure, geometry, and properties must be carefully designed to meet specific requirements such as mechanical strength, controlled degradation, and antibacterial properties. Scaffolds for bone tissue need to be robust due to the bone’s stability role, while those for soft tissues like muscles and tendons should be flexible and resilient to match their elasticity.
All materials applied to the environment of the living organism must pass a series of biocompatibility tests. For instance, implants are scaffolds that have direct contact with blood, tissues, membranes or skin, are subjected to cytotoxicity, blood compatibility, carcinogenicity, biodegradation, sensitization and reactivity with cells.
Drug delivery scaffolds are advanced tools that enhance the therapeutic benefits of drugs by ensuring targeted and controlled delivery to specific tissues or organs.
One essential criterion impacting the capacity of polymeric materials to function as scaffolds is the content’s ease and rate of release relative to their degradation mechanisms. Optimal performance requires retaining the molecules within the delivery scaffold to be released at an appropriate rate to match the healing or growth rate of the tissue.
The delivery method must consider the drug’s pharmacokinetics and pharmacodynamics.
Material properties and composition of tissue-engineered replacement scaffolds can play an important part in driving function for specific disease applications. Indeed, a balance between mechanical properties and architecture known to be conducive is needed to enable tissue regeneration.
When used in a three-dimensional context, these materials are processed into microporous and/or nanoporous cell carriers of various structures and properties.
Noteworthy, injectable polymers such as polyesters, poly-glycolic acid, and poly-caprolactone are biodegradable and biocompatible materials that are usually the preferred choice for localized drug delivery of therapeutic agents, especially into tumors and regenerative medicine, such as infected tissues. They offer tunable and sustained drug release, reducing surgical needs. The applications include tissue fillers, drug carriers, and scaffolds for tissue regeneration.
The use of biodegradable synthetic polymers
Polymeric materials may be divided into natural and synthetic. Although they differ in origin, their functions are similar. Natural polymers, such as collagen, cellulose, chitosan, and hyaluronic acid (HA), are being considered as potential scaffolds for tissue regeneration of different tissues, including, but not limited to, bone, cartilage, and skin. Synthetic polymers such as polycaprolactone (PCL) and poly lactic-co-glycolic acid (PLGA) are gaining popularity. They have also been evaluated for their functionality as scaffolds in regenerative medicine [2].
PLGA is a versatile, biodegradable, and biocompatible copolymer approved by the FDA and EMA. It can be customized in terms of molecular weight and copolymer ratios to suit specific applications. PLGA is soluble in a variety of solvents, including organic solvents such as chloroform, acetone, ethyl acetate and tetrahydrofuran. It can also be processed into various shapes and sizes, and allows for controlled drug release.
When combined with nanotechnology, PLGA’s unique properties enable a wide range of biomedical applications. It can encapsulate both organic and inorganic materials, including small-molecule drugs, vaccines, proteins, and nanoparticles. This adaptability makes PLGA an ideal drug delivery system (DDS) for both hydrophobic and hydrophilic molecules.
Moreover, combining PLGA with inorganic nanoparticles (INPs) to create nanocomposite materials can enhance its applications while maintaining low toxicity. PLGA scaffolds are also effective in bone tissue regeneration [3], helping to correct orthopedic defects, treat bone neoplasia, and aid recovery after surgery.
Porous silk scaffolds, which are natural polymers, cannot be used alone because they have a long degradation rate, which makes it difficult for them to be replaced by the surrounding tissue. Hybrid scaffolds combined with synthetic polymers, such as PLGA, for a tunable degradation rate and hydroxyapatite nanoparticles (Hap NPs) can offer beneficial attributes for optimized bone tissue engineering [4].
In summary
With the growing evidence on the efficacy of biodegradable polymers, they reveal valuable utilization for the controlled drug delivery and tissue engineering. Moreover, these smart drug delivery systems can cross biological barriers and deliver multiple drugs simultaneously, providing prolonged and controlled drug release at the target site. Their application plays a crucial role in minimizing drug toxicity, increasing bioavailability, and reducing dosage frequency.
Biodegradable polymers are highly valued in regenerative medicine due to their ease of application and biocompatibility. They can be combined with various signaling molecules and cells, enhancing their potential as scaffold materials. There is growing interest in developing scaffolds that act as biomatrices for active pharmaceutical ingredients with improved release properties.
3D bioprinting, which uses polymeric biomaterials as bio-ink, is an evolving technology that allows for flexible, on-demand, and cost-effective production of customized drug delivery systems. Additionally, nanostructure-based systems, such as liposomes and nanoparticles, help overcome solubility issues and deliver small molecules to their target sites while minimizing off-target side effects.
At SEQENS, we develop and manufacture GMP and non-GMP polymers for drug delivery, medical device and life science applications.
[1] Mansour A. Drug Delivery Systems in Regenerative Medicine: An Updated Review. Pharmaceutics. 2023, 15, 2, 695.
[2] Aoki, K.; Saito, N. Biodegradable Polymers as Drug Delivery Systems for Bone Regeneration. Pharmaceutics 2020, 12, 95.
[3] a) Gentile, P. et al. An Overview of Poly(Lactic-Co-Glycolic) Acid (PLGA)-Based Biomaterials for Bone Tissue Engineering. Int. J. Mol. Sci. 2014, 15, 3640–3659; b) Ferrone, M.L.; Raut, C.P. Modern Surgical Therapy: Limb Salvage and the Role of Amputation for Extremity Soft-Tissue Sarcomas.
Surg. Oncol. Clin. N. Am. 2012, 21, 201–213 ; c) Martou, G.; Antonyshyn, O.M. Advances in Surgical Approaches to the Upper Facial Skeleton. Curr. Opin. Otolaryngol. Head Neck Surg. 2011, 19, 242–247.
[4] Sheikh FA. et al. Hybrid scaffolds based on PLGA and silk for bone tissue engineering. J Tissue Eng Regen Med. 2016 Mar;10(3):209-21.
info.cdmo@seqens.com