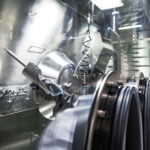
HPAPI – from early development to full-scale manufacturing
Highly potent drugs represent a growing proportion of medicines, including therapies in development and those commercially available. HPAPIs offer a range of benefits over conventional Active PharmaceuticaI Ingredients, including high target specificity, retention (in their active form) within the body for longer durations and fewer side effects.
At present, more than 40% of all drugs are highly potent in nature. Furthermore, 60% of HPAPIs are in the oncology field, and as approximately one third of all new drug approvals are currently cancer medicines, which represents a substantial market opportunity. Other therapeutic areas where drugs may be highly potent include asthma, diabetes, cardiovascular disease, hormone imbalances and autoimmune disorders. The demand for the capability and capacity to manufacture HPAPIs, particularly for CDMOs, is rising significantly. From lab to commercial scale, this article looks at some key considerations and best practices that are required for implementing the handling and manufacturing of these compounds.
In this article, discover some best practices and synthetic route considerations for delivering highly potent drugs.
> Discover the full article on Specialty Chemical Magazine <
Risk analysis
The EU regulatory guideline came into effect for all new pharmaceutical products on June 2015, and for all existing pharmaceutical products on December 2015. This guideline centres on the use of the acceptable daily exposure (ADE) and the operational considerations associated with implementation.1
The ADE is defined as the dose of an active pharmaceutical ingredient PI unlikely to cause adverse effects if an individual is exposed, by any route, at or below this dose every day over a lifetime. It is considered synonymous with the term permitted daily exposure (PDE).
The guidance states that APIs require an ADE. Hence, other substances such as starting materials, process intermediates and cleaning agents may benefit from an ADE. Problems in setting ADEs for these additional substances typically relate to toxicological data limitations precluding the ability to establish a formal ADE.
Established methodologies, such as occupational exposure limits (OELs) or bands (OEBs), can be used or adjusted as the availability of data for the API will increase throughout the drug development lifecycle. A compound is deemed to be potent in pharmaceutical terms if it has an eight-hour, time-weighted average OEL of ≤10 µg/m3 (Figure 1).
An OEB is a process intended to accurately assign chemicals into specific categories (bands), each corresponding to a range of exposure concentrations designed to protect worker health. These bands are assigned based on a chemical’s toxicological potency and the adverse health effects associated with exposure to it.2
Challenges for industrialisation
During process development studies, several technical challenges need to be addressed, due to the highly potent and potentially toxic nature of these substances. These include:
- Containment: HPAPIs must be handled in a highly controlled environment with stringent containment measures to prevent exposure to workers, the environment and the product itself
- Cross-contamination: There is a risk of cross-contamination when manufacturing HPAPIs because they are typically manufactured in the same facility as other products. This can occur during equipment cleaning, transfer operations and raw material handling
- Solubility: HPAPIs may have poor solubility, which can make it difficult to develop stable and effective formulations
- Analytical testing: Analysing HPAPIs requires specialised analytical methods and equipment that can accurately detect and quantify trace amounts of the substance. Indeed, the required specifications of potential genotoxic impurities are in the range of ppm or ppb
- Scale-up: Scaling up the manufacturing process for HPAPIs can be challenging due to the need for precise control and containment measures
- Engineering controls: Engineering controls such as air handling systems and ventilation can reduce the concentration of HPAPIs in the air. These controls can also help prevent cross-contamination and maintain product quality
Overall, manufacturing an HPAPI requires specialised equipment, expertise and strict control measures to ensure product quality and safety. Once the planning of measures has been defined and implemented, strict, rigorous and precautionary measures will need to be taken continuously in order to guarantee full control of high potency manufacturing.
Conclusion
The manufacturing of HPAPIs requires an adequate working environment (to prevent cross contamination within multi-product assets), stringent manufacturing protocols (to comply with the established regulatory standards) and a trained workforce (to handle highly potent materials satisfactorily).
Chemical process development that optimises safety and efficiency from lab-scale to full commercial production, along with appropriate process equipment, the use of advanced cleaning and trace analytical testing methods, are all key considerations. It requires comprehensive management systems, including industrial hygienists, maintenance teams, quality assurance experts, operations teams, and engineering and automation personnel for risk prevention, such as occupational exposure or cross-contamination.
In addition, it requires an expensive infrastructure, which is often complex to engineer, install and maintain. Track record experience in manufacturing through a broad technology portfolio, excellence in process engineering and safety standards are the ultimate criteria.
For these reasons, many pharmaceutical companies rely on third party service providers to leverage their technologies for manufacturing highly potent and achieve greater operational flexibility. The essential expertise of integrated CDMO is believed to be capable of enabling reduction in the time-to-market a product and offer significant cost-benefits.
References:
- E.P. Hayes, R.A. Jolly, E. Faria, E.L. Barle, J. Bercu, L. Molnar, B. Naumann, M. Olson, A. Pecquet, R. Sandhu, B.K. Shipp, R. Sussman, P. Weideman, Regulatory Toxicology & Pharmacology (2016), doi: 10.1016/j.yrtph.2016.06.001
- The NIOSH Decision Logic for OEBs, The Synergist, American Industrial Hygiene Association, March 2016
Juliette Martin, Scientific Communication Manager at SEQENS